Foundations For Structures: Artic and Subartic Construction - indexChapter 1: Introduction - ufc_3_130_040010Figure 1-1. Meteorological data and ground isotherms, Fort Yukon, Alaska99Figure 1-2. Freeze or thaw penetration vs mean annual temperatureFigure 1-3. Typical temperature gradients under permafrost conditions, Kotzebue Air Force Station, AlaskaFigure 1-4. Typical temperature gradients in the ground Wind and other factorsTable 1-1. Stages of Relative Human Comfort and the Environmental Effects of Atmospheric CoolingChapter 2: Basic Considerations Affective Foundation DesignFigure 2-1. Thermal conductivity vs density and porosity of typical materialsSeasonal frost heave and settlementFigure 2-2. Ice wedges in polygonal ground areaGroundwater - ufc_3_130_040021Figure 2-3. Moisture content changes caused by freezing40. Water available at base of specimen during testFigure 2-5. Plan and elevation of surcharge field experiment, Fairbanks, Alaska28Effect of surchargeFigure 2-7. Heave vs frost penetration for various total stresses, surcharge field experimentFigure 2-8. Comparison of laboratory and field measurements of effects of surcharge Foundation materialsFigure 2-9b. Maximum Frost Heave PressuresFigure 2-9c. Maximum Frost Heave Pressures. (Grain, Size Distribution, of Soils.)Figure 2-10. Summary of maximum stress in compression vs temperatureFigure 2-11a. Summary of Soil Characteristics34 (Gradations)Figure 2-11b. Summary of Soil Characteristics (Void Ratio vs Coefficient of Permeability)Figure 2-11c. Summary of Soil Characteristics. (Soils Test Data)Figure 2-12. Summary of maximum stress in tension vs temperatureFigure 2-13. Summary of maximum shear stress of frozen soil vs temperatureFigure 2-14. Effect of rate of stress application on failure strengthFigure 2-15. Plastic deformation of frozen soils under constant compressive stressFigure 2-16. Compressive strength vs ice content, Manchester fine sandFigure 2-17. Longitudinal and torsional wave velocity, dynamic moduli of elasticity and rigidityIce masses in cleanFigure 2-18. Typical record of ice in permafrostStructural materialsStructural materials -Cont.Table 2-2. Specific Gravity and Strength of WoodFigure 2-19. Influence of moisture and temperature on strength of woodFigure 2-20. Effect of temperature on strength of woodFigure 2-21. Toughness shown in flexure testFigure 2-22b. Effect of Temperature on Flexure Properties and of Temperature and Moisture Content on Modulus of Elasticity of WoodConcrete and masonryFigure 2-23b. Effect of Concrete Mix and Moisture Conditions on Strength of Concrete at Low TemperaturesThermal insulating materialsFigure 2-24. Moisture absorption of insulation board by soaking in waterFigure 2-25b. Internal moisture distribution in insulation board under different test conditionsFigure 2-25c. Internal moisture distribution in insulation board under different test conditionsFigure 2-25d. Internal moisture distribution in insulation board under different test conditionsTable 2-3. Moisture Distribution in Cellular Glass after 20 Years Burial in the Annual Frost Zone, Fairbanks, Alaska"Chapter 3: Site Investigations Detailed direct site explorationFigure 3-1. Required extent of explorations for large structureClimatic dataPhysiography and geologyFigure 3-2. Typical exploration logFigure 3-3. Dry density and water content vs. depth for a soil exploration in permafrostFigure 3-4. Thaw-consolidation test on undisturbed sampleFigure 3-5. Consolidation test results for undisturbed samples from two drill holes at same siteFigure 3-7. Estimated settlement vs. depth of thaw for different explorations at the same timeThermal propertiesFrost heave field observationsFigure 3-8. Pattern of cracks in taxiway pavement, Elmendorf AFB, AlaskaFigure 3-9. Soil profile, south wall of trench near south edge of taxiway, Elmendorf AFB, AlaskaChapter 4: Foundation DesignFigure 4-2. Design alternativesFigure 4-3. Thawing of permafrost under 3 story, reinforced concrete, 500-man barracks, Fairbanks Selection of foundation typeModification of foundation conditions prior to construction Simplified example of selection of foundation type in an area of continuous permafrost Simplified example of selection of foundation type in an area of continuous permafrost -Cont.Table 4-1. n - Factors for Freeze and Thaw (Ratio of Surface Index to Air Index)Figure 4-4a. Approximate depth of thaw or freeze vs air thawing or freezing index and n-factor for various homogeneous soilsFigure 4-4b. Approximate depth of thaw or freeze vs air thawing or freezing index and n-factor for various homogeneous soilsDesign depth of thaw penetrationFigure 4-6a. Thaw vs time. Canadian locations (after Sebastyan188)Estimation and control of thaw or freeze beneath structures on permafrostFigure 4-7. Depth of thaw vs air thawing index for unpaved surfacesFigure 4-8. Thaw depth as affected by runway construction on permafrostFigure 4-9. Effect of heated structure size on depth and rate of thawTable 4-2. Thaw Penetration Beneath a Slab-on-Grade Building Constructed on PermafrostFigure 4-10. Degradation of permfrost under five-story reinforced concrete structure, Fairbanks, Alaska. FoundationFigure 4-11. Permafrost degradation under 16-feet-square heated test buildings without air space, beginning at end of constructionFigure 4-12a. Typical foundation thaw near Fairbanks, AlaskaVentilated foundationsFigure 4-14. Foundation in permafrost area for men's barracks, Thule, GreenlandFigure 4-15. Post and pad type foundation for composite building, Fort Yukon, AlaskaFigure 4-16. Footing on permafrost foundation, Bethel, AlaskaFigure 4-17. Footings on permafrostFigure 4-18. Typical ventilated foundation design for structure supported on pilesFigure 4-19. Woodpile foundation for small residences, Fairbanks, AlaskaFigure 4-20. Typical pile foundation for light utility building, Fairbanks, AlaskaFigure 4-21. Steel pile foundation for utility building showing sunshade, Bethel, AlaskaFigure 4-22. Foundation for men's club, Thule, GreenlandFigure 4-23. Two story steel frame building on footings and piers at Churchill, Manitoba, CanadaFigure 4-24. Utility and maintenance building, Fairbanks, Alaska (by CRREL)Figure 4-25. Ducted foundation for garage, Fairbanks. AlaskaFigure 4-26. Pan duct foundation, Thule, GreenlandFigure 4-27a. Typical Pan Duct Foundation, Showing Section at Plenum chamber and at Interior Column for WarehouseFigure 4-27b. Typical Pan Duct Foundation, Showing Section at Plenum Chamber and at Interior Column for WarehouseFigure 4-28a. Foundation Details and Maximum Thaw Penetration for Selected Years, Hangar at Thule, Greenland (Deep air duct foundation detailsFigure 4-28c. Foundation details and maximum thaw penetration for selected years, hangar at Thule, GreenlandVentilated foundations -Cont. - ufc_3_130_040109Ventilated foundations -Cont. - ufc_3_130_040110Figure 4-29. Schematic of ducted foundationVentilated foundations -Cont. - ufc_3_130_040112Figure 4-30. Properties of dry air at atmospheric pressureVentilated foundations -Cont. - ufc_3_130_040114Foundation insulationInsulation of ventilated and ducted FoundationsFigure 4-32. Flow net, concrete slab on grade, insulatedFigure 4-33. Flow net, extrapolated from field measurements, with 1-1/2-inches cellular glass insulationFigure 4-34. Floor temperature 6 in. from wall vs daily average air temperature measured at Loring AFBFigure 4-35. Comparison of predicted and measured floor temperatures for a concrete slab on gravel with vertical insulationFigure 4-36a. Thermal resistance vs design winter temperature for various vertical lengths of insulation for kitchens and mess hallsFigure 4-36b. Thermal resistance vs design winter temperature for various vertical lengths of insulation for Kitchens and Mess HallsFigure 4-36c. Thermal resistance vs design temperature for various vertical lengths of insulation for Kitchens and Mess HallsFigure 4-37a. Thermal resistance vs design winter temperatures for two vertical lengths of insulation for barrack buildingsFigure 4-37a. Thermal resistance vs. design winter temperatures for two vertical lengths of insulation for barrack buildingsTable 4-3. Recommended Perimeter Insulation for Various Design Winter TemperaturesGranular matsProtection against solar radiation thermal EffectsControl of movement and distortion from freeze and thawFigure 4-38a. Wood frame residence 32 x 32 feet on rigid concrete raft foundation, Fairbanks, AlaskaFigure 4-38c. Wood frame residence 32 x 32 feet on rigid concrete raft foundation, FairbanksFigure 4-39a. Wood frame residence 32 x 32 feel on wood pile foundation, FairbanksFigure 4-39b. Wood frame residence 32 x 32 feet on woodpile foundation, Fairbanks, AlaskaFigure 4-40b. Wood frame garage 32x 32 feet on rigid concrete raft foundation Fairbanks, AlaskaFigure 4-40c. Wood frame garage, 32x 32 feet on rigid concrete raft foundation, Fairbanks, AlaskaControl of settlements which may result from thawFigure 4-43. Average heave vs. time. Floors of unheated wing hangars, Loring AFB, Limestone, MaineControl of frost heave and frost thrustFigure 4-44. Test observations, 1962-63, 8-inch steel pipe pile, placed with silt-water slurry in dry-augered holeFigure 4-45. Test observations, 1962-63, creosoted timber pile, average diameterControl of frost heave and frost thrust -Cont.Allowable design stresses on basis of ultimate strengthFigure 4-47. Frozen soil creep tests, unconfined compression, Manchester fine sandFigure 4-49. Unconfined compression creep curves for frozen Ottawa sand at 29FFigure 4-50. Ultimate strength vs. time to failure for Ottawa sand (20-30 mesh) at various temperaturesFigure 4-51. Mohr's envelopes for frozen sand (Ottawa sand, 20 - 30 mesh)Allowable design stresses on basis of ultimate strength -Cont.Figure 4-52. Stress and time to failure in creepTable 4-4. Allowable Design Cohesive Stress for Saturated Frozen Soil in T/ft2 on Basis of Ultimate StrengthFigure 4-53. Mohr envelopes for frozen soils under moderately rapid loading, from tension and unconfined compression TestsEstimation of creep deformationTable 4-5. Constants for Equation 4 Dynamic loadingFigure 4-55. Frozen soil creep tests on Manchester fine sand, unconfined compressionDetermination of response characteristics of foundation materialsLow stress, vibratory loadsTable 4-6. P-wave Velocities in Permafrost (after Barnes"' with some Additions)Figure 4-56. Complex dynamic Young's modulus vs. volume ice/volume soil ratio for frozen saturated, non-plastic soilsFigure 4-57. Complex dynamic shear modulus vs. ice saturationFigure 4-58. Complex dynamic Young's modulus vs. ice saturationFigure 4-60. Complex dynamic shear modulus vs. frequency at constant dynamic stressDesign of footings, rafts and piersFigure 4-61a. Bearing capacity formulas. (Ultimate bearing capacity of shallow foundations under vertical centric loads)Figure 4-61b. Bearing capacity formulasIllustrative examples for the design of footings in permafrostFigure 4-62. Boring log and soils condition (by CRREL)Figure 4-63. Case of isolated square footing (by CRREL)Illustrative examples for the design of footings in permafrost -Cont. - ufc_3_130_040168Figure 4-64. Vertical stress at centerline (by CRREL)Illustrative examples for the design of footings in permafrost -Cont. - ufc_3_130_040170Table 4-7. Computation of Temperature Below Top of PermafrostFigure 4-65. Temperature distribution for permafrost below footingCreep settlement computationFigure 4-66. Conditions for creep analysisTable 4-8. Settlement ComputationsFigure 4-67. Unconfined compression creep testFigure 4-68. Conditions for bearing capacity analysis of square raft (by CRREL)Table 4-9. Stress Distribution Beneath the Uniformly Loaded AreaFigure 4-69. Frozen soil column - diagrams of temperature and stress distributionTable 4-10. Settlement Computations (by CRREL)Concrete pilesPile emplacement methodsInstallation in bored holesInstallation by steam or water thawingFigure 4-70. Latent heat of slurry backfillNatural freezebackFigure 4-71. General solution of slurry freezeback rateArtificial freezebackFigure 4-73. Natural freezeback of piles in permafrost during winter and summerFigure 4-74. Compressor for artificial freezeback of pilesFigure 4-75. Refrigeration coils on timber piles for artificial freezebackPeriod of installationFigure 4-76. Influence of slurry on temperature of permafrost between pilesHeat transfer by thermal pilesHeat transfer by thermal piles -Cont. Single-phase piles Single-phase piles -Cont.Figure 4-77. Stresses acting on piling for typical permafrost conditionFriction pilesFigure 4-79. Load, strain and stress distribution for pile in permafrost with zero load at tipFriction piles -Cont. - ufc_3_130_040201Figure 4-80. Load test of steel pipe pileFigure 4-81. Load distribution along pile during test, strain-gage instrumented pileFigure 4-82. Tangential adfreeze bond strengths vs. temperature for silt-water slurried Friction piles -Cont. - ufc_3_130_040205Figure 4-83b. Example of computation of sustainable load capacity of pile in permafrostFigure 4-83c. Example of computation of sustainable load capacity of pile in permafrostFigure 4-83d. Example of computation of sustainable load capacity of pile in permafrostFigure 4-83d. Example of computation of sustainable load capacity of pile in permafrost -Cont.Load testing of piles in permafrostFigure 4-84. Load-settlement test. 1O-kip incrementsFigure 4-85. Effect of rate of loading and temperature on adfreeze strength of steel pipe pilesFigure 4-86. Load settlement test. single incrementWalls and retaining structuresFigure 4-87. Walls and abutmentsFigure 4-88. Thickness of non-frost-susceptible backfill behind concrete wallsTower foundationsFigure 4-89. Granular pad tower foundationFigure 4-90. Foundation designs employing minimum or no NFS granular borrowed material (both are frost-susceptible foundation)Figure 4-91. Effects of granular mats on frost penetration and heaveTower foundations -Cont.Bridge foundationsFigure 4-92a. Geologic sections at two Alaska railroad bridges in Goldstream Valley near Fairbanks, AlaskaFigure 4-92b. Geological sections at two Alaska railroad bridges in Goldstream Valley near Fairbanks, AlaskaCulvertsAnchoragesFigure 4-94. Failure planes for batter and vertical anchor installationFoundations for non-heated facilitiesExterior footings and pilesConnection of utilities to buildingsFigure 4-96. Exterior apron designFigure 4-97b. Utility connections to buildings. (Lateral utility connections.)Drainage around structuresStability of slopes during thawFigure 4-98. Slopes in frost and permafrost areasSloughing and thaw settlement in areas of permafrostFigure 4-99. Ice exposed in vertical cut for Trans-Alaska Pipeline Access Road between LivengoodFigure 4-100. Slope resulting from melting of ice in vertical cut face during one summer, Trans-Alaska Pipeline Access Road between Livengood and the Yukon RiverChapter 5: Survey Datum PointsFigure 5-1. Recommended permanent benchmarkTemporary datum pointsChapter 6: Construction ConsiderationsExcavationEmbankment and backfillPlacing concrete under freezing air or ground temperatureAir entraining agentsHigh early strength cementMixing, transporting and placingProtection after the curing periodTable 6-2. Insulation Requirements for Concrete Walls and for Concrete Slabs and Canal Linings Placed on the GroundTable 6-2. Insulation Requirements for Concrete Walls and for Concrete Slabs and Canal Linings Placed on the Ground -Cont.Protection against the environmentFoundation protectionChapter 7: Monitoring PerformanceThermocouplesSoil electrical resistanceMonitoring groundwaterFigure 7-1b. Permafrost and frost probing techniques. (Probing through frozen ground.)Figure 7-2. Electrical resistance gage for determination of frost penetration (by CRREL)Appendix A: References - ufc_3_130_040260Appendix A: References -Cont. - ufc_3_130_040261Appendix A: References -Cont. Appendix A: References -Cont. - ufc_3_130_040263Appendix A: References -Cont. - ufc_3_130_040264Appendix A: References -Cont. - ufc_3_130_040265Appendix A: References -Cont. - ufc_3_130_040266Appendix A: References -Cont. - ufc_3_130_040267Appendix B: Bibliography - ufc_3_130_040268Appendix B: Bibliography -Cont.
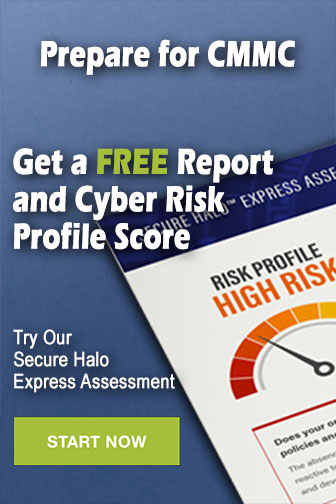